Roger Best and Chris Sataua
Will the pole vault world record fall in the 2016 Rio Summer Olympics? That question will draw a diverse set of opinions, and we will make our prediction at the end of this short research paper. But, to understand our prediction for the world record in Rio, we need to look at how the pole vault event has evolved over time and what role sports product innovation has played in setting 106 world records over the past 166 years.
Let's start with a simple question: If a world-class sprinter and a world-class pole-vaulter ran a 100-meter race, we would expect the sprinter to win. But if each carried a 17-foot pole weighing 7 pounds, who would win?
An elite pole vaulter can reach a top speed of 20 to 22 miles per hour. But could an elite sprinter match or exceed this speed carrying a 17-foot pole weighing 7 pounds?
The pole vault is one of the most athletically complex and demanding of all sports competitions. It requires the:
- Speed of a sprinter to create the energy needed to execute the vault
- Takeoff of a long jumper to achieve maximum takeoff energy and angle
- Upper body strength of a shot putter to transfer energy from body to the pole
- Timing and agility of a gymnast to swing up and execute a body invert
- Body control of a ballerina to arch, roll, clear the bar, and fall to the ground without injury.1
Archeological evidence shows that the ancient Egyptians used poles to scale enemy walls as early as 2,500 B.C.2 About 900 years later (1829 B.C.), pole vaulting evolved into a sport from the use of poles by Celtic farmers to vault across streams and irrigation ditches. The origin of the sport explains why the objective during its early history was distance rather than height.3 It was not until the 1800s that the pole vault became a track-and-field event where athletes competed for height.4 The equipment was primitive in that the pole was made of wood and the running surface was typically grass or dirt. There was no pole box or cushioned landing area. Footwear and apparel were the same as those used for other track-and-field events. Up until the late 1800s, athletes were allowed to climb up the pole in order to reach a higher point before pushing off.5 As shown in Exhibit I, the pole vault world record has been broken 106 times over the 165 years between 1850 and 2015.
Exhibit I – World Records and Pole Performance Eras
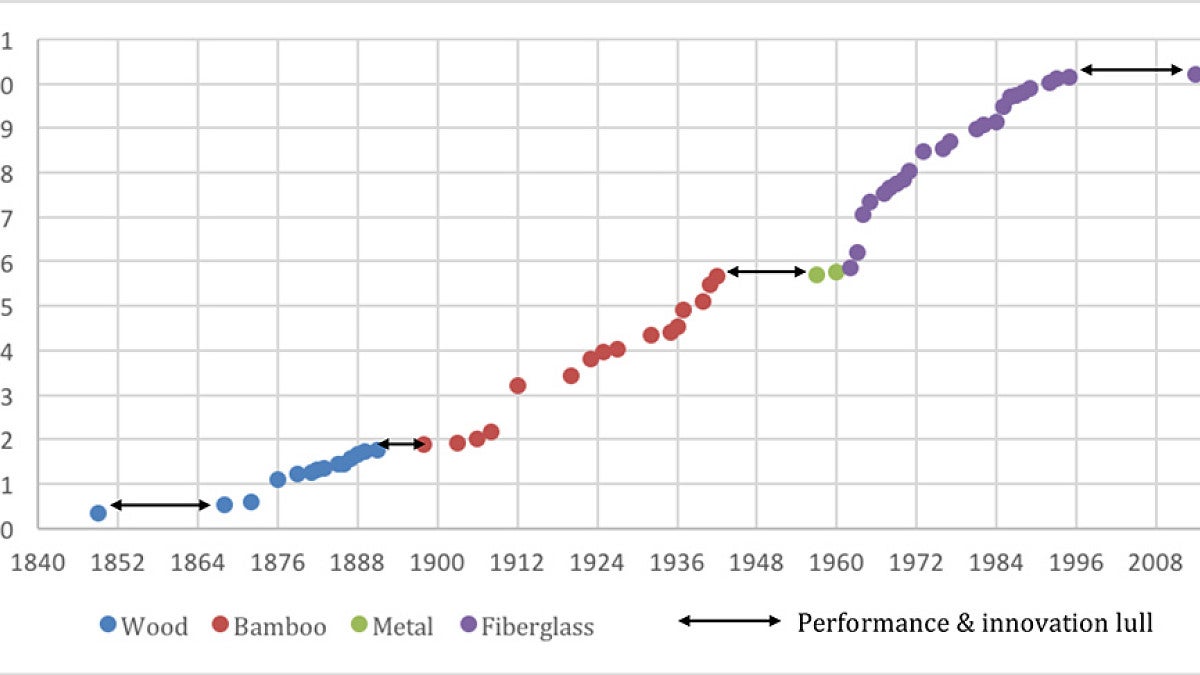
Exhibit II – Pole Vault World Records and Product Innovations
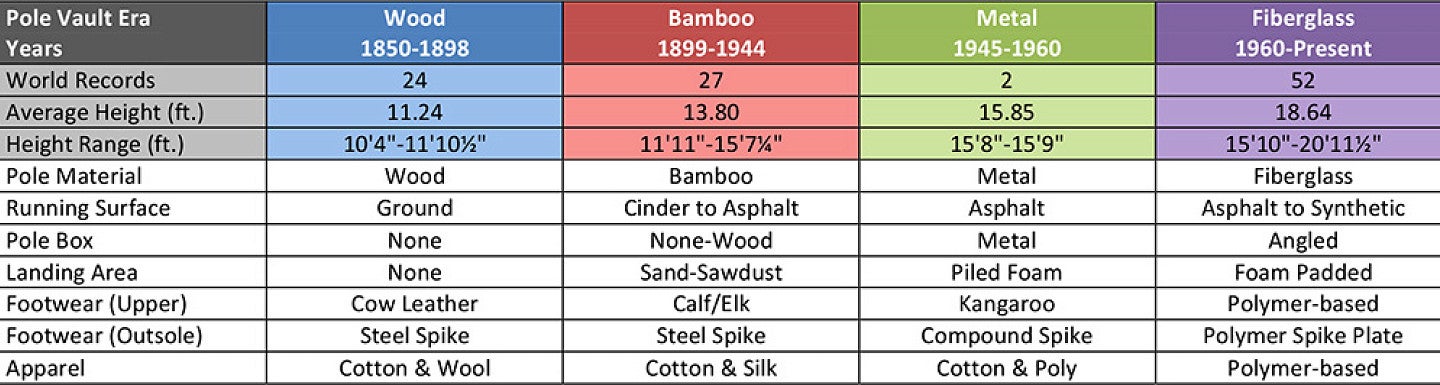
Wood era: From 1850 to 1898, poles were generally ash or hickory.6 British vaulters produced all but one of the 24 world records that were set during this 48-year period. The height of world-record vaults during the wood era increased 1"18½", as shown in Exhibit I. But by 1898, the wood pole, footwear, apparel, and techniques that were common among vaulters had reached the point of diminishing gains in the quest for new world records. Innovators began to experiment with alternatives to the heavy ash and hickory poles, resulting in the lighter and more flexible bamboo pole. This created a discontinuous innovation as the sport moved from a wood pole (an old technology) to bamboo pole (a new technology). As shown in Exhibit II, this coincided with other discontinuous innovations in footwear, apparel, running surface, and the pole box and landing area.
Bamboo era: With the introduction of bamboo poles, new world records were once again set more frequently. During the bamboo era, from 1899 to 1944, 20 athletes set 27 world records. Over this span of 45 years, the world record improved by 3"9". The tremendous increase in height was due to the lighter bamboo pole and to product innovations in the running surface, pole vault footwear, and apparel, all of which enabled vaulters to improve their approach speed—for world-class vaulters, no other factor is more important. The bamboo era also saw the introduction of the pole box and the cushioned landing area.
Metal era: In the late 1940s, the focus was on yet a lighter pole that would further improve a vaulter's approach speed. Product innovators developed tubular steel and aluminum poles. The metal era lasted only 15 years, and only two world records were set, adding less than 2" to the record. With less than spectacular results, product innovators began their pursuit of a glass-fiber-reinforced polymer composite (GFRP) pole.
Fiberglass era: The first world record set with a fiberglass pole occurred in 1960. The GFRP pole is lighter than the poles of previous eras, and it is highly flexible, which provides greater energy transfer from the athlete to the pole. The pole's flexibility also contributes to higher vaults by enabling athletes to propel feet first into the air and execute a vertical handstand before releasing the pole. This era also coincides with polymer-based product innovations in running surface, footwear, and apparel. Together, the fiberglass pole and other polymer innovations have contributed to 53 world records since 1960, and the record has increased by almost 4"6". But in the past 21 years, the record has been broken only one time, by less than an inch.
Pole Vault Physics and Performance
The vault height achieved boils down to the physics of the pole vault and the creation, transfer, and dissipation of energy as illustrated in Exhibit III.7 At the start, the vaulter's kinetic energy is zero. During the runway approach, kinetic energy increases exponentially with greater speed.
Approach speed: The approach speed of elite vaulters is highly correlated with the height of the vault, as shown in Exhibit III. The referenced study found that approach speed accounted for 67% of the height achieved. During the approach, vaulters generate kinetic energy in proportion to their weight and speed-squared. The equation below shows the kinetic energy Sergey Bubka created when he set his last world record of 20"1¾" (6.14 meters) in 1994.
Exhibit III – Pole Vault Physics: Energy Creation, Energy Transfer, and Gravitational Energy
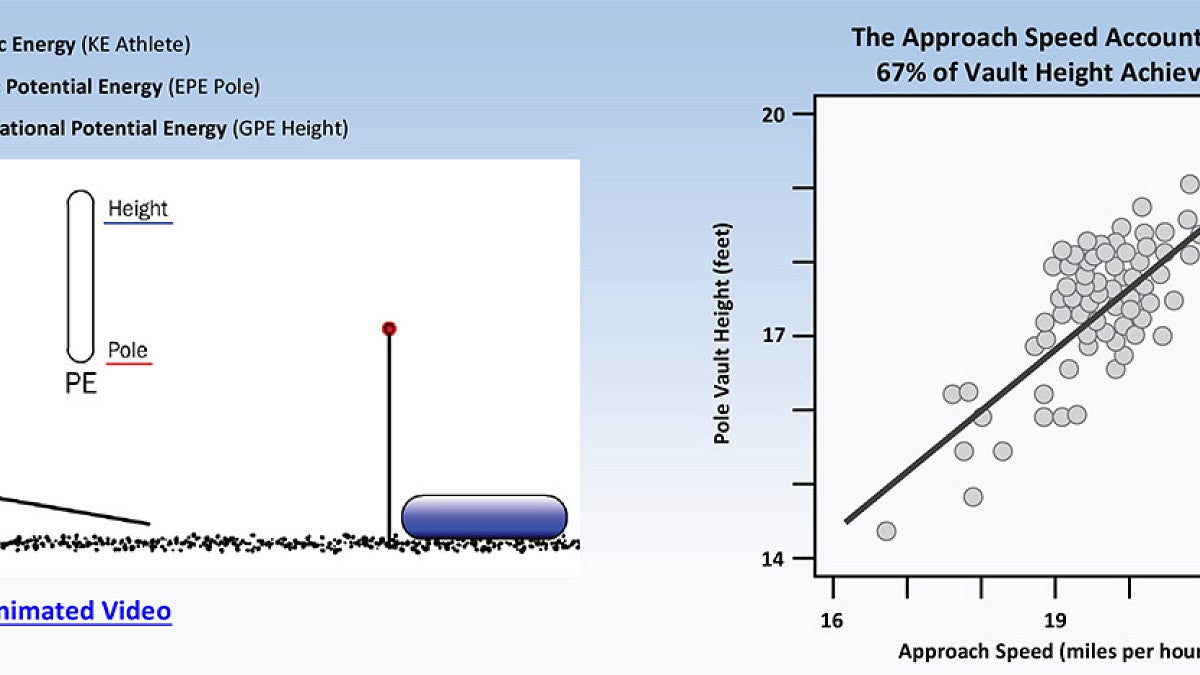

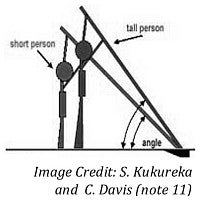
Bubka's total mass was 5.75 based on his weight of 178 pounds and a pole weighing about 7 pounds, resulting in a total weight of 185 pounds.9 At the time of his pole plant and takeoff, his speed was about 22.3 miles per hour (32.8 feet/second).10 His mass and speed created 3,137.5 foot-pounds of kinetic energy.
Takeoff: At the point of pole plant and takeoff, the kinetic energy created during the approach run (green in Exhibits III and IV) is transferred to the pole as elastic potential energy (red in the Exhibits III and IV). The fiberglass pole greatly increases the amount of energy transferred to the pole, but the takeoff angle also impacts this energy transfer. The angle should be as high as possible since the higher the takeoff angle, the greater the amount of kinetic energy that is converted into vertical energy. Taller pole vaulters have an advantage here: with the same pole grip height as a shorter vaulter, the taller vaulter will have a higher takeoff angle, as shown in the illustration at the right.11
Swing up: This is where upper body strength is a difference maker. In the swing up, vaulters swing their trail leg forward and row the pole, bringing the top arm down to the hips, while keeping the trail leg straight to store more potential energy in the pole.12 The rowing motion also keeps the pole bent for a longer period of time, allowing the vaulter to attain the optimum, 90-degree position.13 The fly away involves the vaulter pushing off the pole so that it falls away from the cross bar as the vaulter goes over the bar facedown. During this stage of the vault, the elastic potential energy captured in the pole is transferred to gravitational potential energy (blue in the Exhibits III and IV).
Fly away: At peak height, the kinetic energy created equals the gravitational potential energy transferred. Because mass appears as a factor on both sides of the equation, they cancel one another out as shown below. Thus, the height due to energy creation is equal to ½ ⋅ (Speed Squared)/Gravity. For Sergey Bubka's last record-breaking vault, we would have predicted a height of 16"8" based on a speed at takeoff of 22.3 miles per hour (32.8 ft./second), as shown below:
Exhibit IV – Pole Vault Physics and Energy Equation
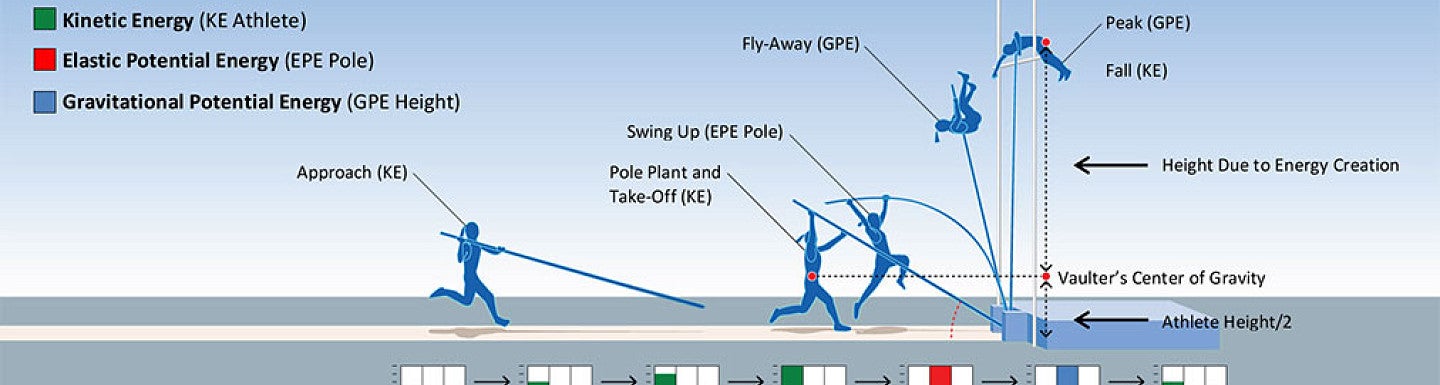

This height is due to the energy created and transferred to height from the center of the vaulter's mass at takeoff and peak. At takeoff, this would be half of the vaulter's height. Sergey Bubka was 6" tall, so the predicted height increases by 3". Additionally, because the vaulter's mass center at the peak is higher than the vaulter's center of gravity, 5" is added, making the overall predicted height 20.09". The actual height achieved was 20".14

The fall: At the peak of the vault, the gravitational potential energy starts to dissipate and transfer to kinetic energy as the vaulter falls to the landing area at the speed of gravity. Upon landing, kinetic energy becomes zero as it is transferred to the landing pads. World-class pole vaulters have vastly improved their training and conditioning regimens over the years, which has undoubtedly helped produce the large number of world records. Additionally, the product innovations shown here contributed to each era's world records shown in Exhibit I.
Approach Speed = F(Athlete) + F(Running Surface, Pole Weight, Footwear and Apparel)
Takeoff Angle = F(Athlete) + F(Pole Length and Pole Box)
Pole Energy Transfer = F(Athlete) + F(Pole Material)
Peak Clearance = F(Athlete) + F(Apparel, Cross Bar and Landing Area)
Product Innovation and S-Curve
Each pole vault era represents a separate discontinuous innovation brought about by a technology shift in materials. A shift from wood to bamboo was a discontinuous innovation in that the old technology (wood) was discontinued and replaced by a new technology (bamboo). Within a pole vault era, continuous innovations in products made of the new materials led to further gains in performance. Continuous innovations typically follow an S-shaped curve. For each of the technology S-curves in Exhibit V, gains in performance grow rapidly in the early periods of a new technology and gradually diminish to smaller incremental gains as the technology's performance limits are being reached.
For example, during the wood pole era innovations in product design (shown in Exhibit II) grew from 1850 to 1890. By 1890 this technology era was reaching its limits, and performance gains over the next 10 years in the world record were minimal until a radical discontinuous innovation occurred with the beginning of the bamboo pole era. As shown in Exhibits I and V, performance gains from 1900 to 1920 were rapid during the early years of this new era of bamboo pole technology.
Exhibit V: Technology and Product Innovation S-Curves
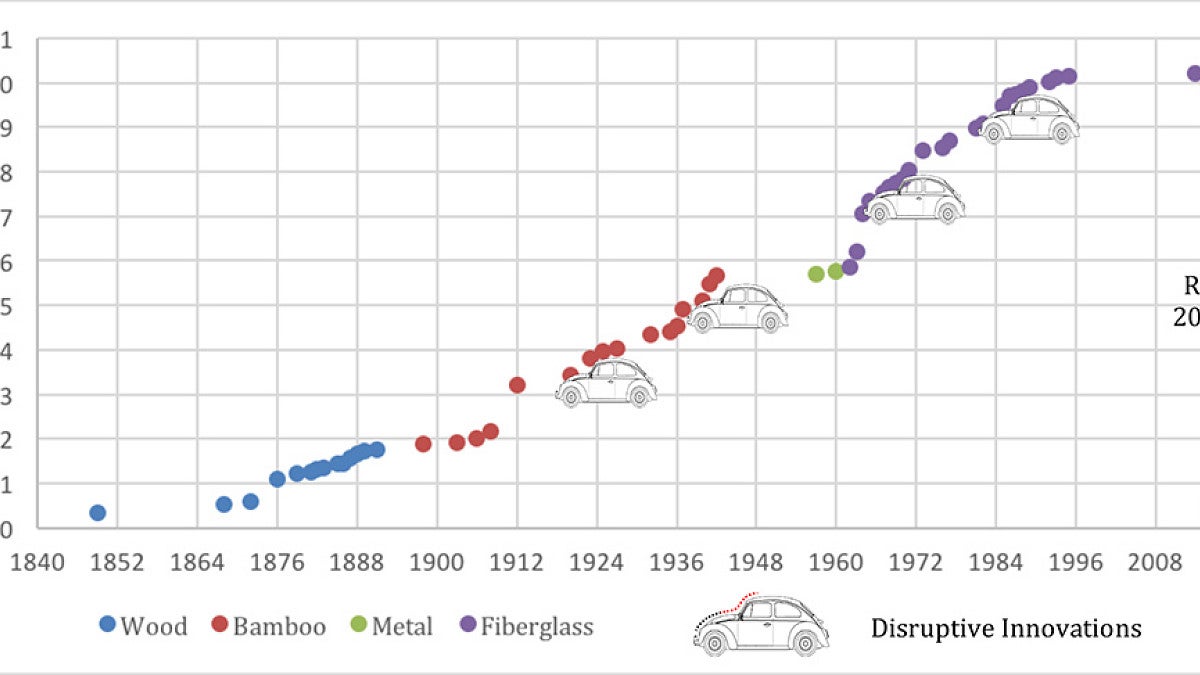
Note that between 1912 and 1920, however, the bamboo S-shaped curve began to flatten and around 1920 started to form a second S-shaped curve within the bamboo pole era. This was due to disruptive innovations within the bamboo era. Innovations in the running surface, footwear, and pole box were disruptive innovations that added a second hump to the S-shaped curve. Disruptive innovations create a disruption of the continuous innovation curve for a specific technology and expand the range of performance.14 The data suggests there was a second disruptive innovation around 1936 that caused a round of new world records between 1936 and 1945.
Two disruptive innovations have also occurred in the fiberglass pole era (as shown in Exhibits I and V) spurred by product innovations in synthetic running surface, polymer footwear and apparel, and other non-pole equipment. Like previous pole technology eras, the fiberglass pole era has reached diminishing gains in world-record performance, with only one new world record set in the past 21 years. If the history of the three previous pole vault eras holds true for the fiberglass era, the flattening of the S-shaped curve points to the end of this era, just as it did for the wood, bamboo, and metal pole material eras.
For a new world record to be set, new materials must enable athletes to run faster to create more kinetic energy and more fully transfer kinetic energy to elastic pole energy. This will require a discontinuous innovation in pole materials, footwear, running surfaces, and pole boxes (as shown in Exhibit V). Athletes will continue to improve and incremental gains in the world record will occur, as was the case at the end of each performance era. Materials development and engineering technology have long been known to have a major impact on pole vault performance. The rules of the International Association of Athletics Federation (IAAF) state:
“A pole may be of any material or combination of materials and of any length or diameter, but the basic surface must be smooth."
Thus, any new pole material, as long as the pole has a smooth surface, would then be permissible. While molecular design (nanotechnology) offers the potential for new pole materials, will the definition extend to microelectronics and the creation of an “intelligent pole" with Intel Inside? Such a pole could alter the takeoff, swing, and push off. If not for competition, it is possible that an intelligent pole could be used in training to provide athlete energy creation and exchange feedback on approach speed, takeoff angle, swing through, and push off. While a new pole material would create discontinuous innovation, an intelligent training pole would create a disruptive innovation to allow world records to grow with the existing pole technology.
Brazil 2016: Will the World Record Fall?
Over the past 20 years, product innovators have looked for ways to improve the fiberglass pole with carbon fiber and woven carbon fiber technologies. Carbon fiber poles, while promising, have not had a role in producing a new world record, but they have been used by vaulters who have set new Olympic records during this period. One thing is clear: the fiberglass pole era has reached its performance limits (as shown in Exhibit V). Taller, faster, and stronger athletes, with improvements in training, conditioning, and technique, will produce new world records with small incremental gains. To reach the performance impact of previous pole vault eras, a discontinuous innovation in the form of a new pole material will be required.
History Favors the Under
In the 2016 Olympics, the world record will be very difficult to break. There have been no major product innovations over the past 20-plus years to inspire product-based performance gains demonstrated in previous pole vault eras. With only incremental improvements in existing pole materials and related product design (continuous innovations), several conditions will have to occur for the world record to fall: (1) the right weather conditions; (2) perfect psychological moment for an elite pole vaulter; (3) an approach speed of more than 22 miles per hour; (4) excellent pole plant and high launch angle of 30 degrees or more; and (5) flawless swing up, fly away, and roll. The odds for this to happen are not good; we are taking the “under" and predicting a new world record will not occur in the 2016 Rio Olympics. However, if a new world record is set, it will be largely due to the athlete, not the pole.
Citations
- Jan Johnson, Russ VerSteeg and Ray Kring, Illustrated History of the Pole Vault, Skyjumpers.com (Norwich, Conn., 2007) p. 6.
- Ibid., p. 7.
- “Ancient Pole Vaulting," Vaulter Magazine, http://vaultermagazine.com/ancient-pole-vaulting/, August 21, 2012, accessed July 15, 2015.
- Johnson, Op. Cit., p. 14.
- “Evolution of the Pole Vault," Vaulter Magazine-Vaulter Club Inc., http://vaultermagazine.com/evolutionof-the-pole-vault/, June 12, 2013, accessed July 15, 2015.
- Ibid.
- Karim Nice, “How Pole Vaulting Works," http://entertainment.howstuffworks.com/pole-vault3.htm, not dated, accessed July 18, 2015.
- Nicholas P. Linthorne and A.H. Gemma Weetman, “Effects of run-up velocity on performance, kinematics, and energy exchanges in the pole vault," Journal of Sports Science and Medicine, June 2012, pp. 245–254.
- Matt Sweet, “This is a world record that is so mythical," Vaulter Magazine, August 2014, p.16. Available online at http://issuu.com/thevaultermagazine/docs/august_final; and “Sergey Bubka," Sports Reference LLC, http://www.sports-reference.com/olympics/athletes/bu/sergey-bubka-1.html, not dated, accessed July 19, 2015.
- Sweet, op. cit.
- Peter M. McGinnis, “Mechanics of the Pole Vault," and Stephen Kukureka and Claire Davis, “The Pole Vault for Engineers."
- “Pole vault," Wikipedia (Article), https://en.wikipedia.org/wiki/Pole_vault, accessed July 18, 2015.
- Ibid
- Venkatesh Rao, “Disruptive versus Radical Innovations," July 23, 2007, Ribbonfarm Blog, http://www.ribbonfarm.com/2007/07/23/disruptive-versus-radical-innovations/
About the Authors
Dr. Roger Best is emeritus professor of marketing at the University of Oregon's Lundquist College of Business and is cofounder of the Sports Product Management Program. Best is the author of Market-Based Management, consultant to many Fortune 500 companies, and creator of many trademarked and patented products. Best is the acting director of the Sports Product Management Research Program.
Chris Sataua is a master's student in the University of Oregon Sports Product Management Program and a Sports Product Management Research Assistant. Sataua has extensive education and experience in design, marketing, and retail management.
The University of Oregon Sports Product Management Program"s mission is to be the worldwide leader in sports product management education and research. The program is built on three pillars of excellence – product innovation, sustainability and global leadership.
This paper is part of the Sports Product Management Research Program. Each year we hope to provide at least one short paper in each of these pillars of excellence.